![]() | ||||||||||||||||||||||||||||||||||||||||||||||||||||||||||||||||||
Osmium | ||||||||||||||||||||||||||||||||||||||||||||||||||||||||||||||||||
---|---|---|---|---|---|---|---|---|---|---|---|---|---|---|---|---|---|---|---|---|---|---|---|---|---|---|---|---|---|---|---|---|---|---|---|---|---|---|---|---|---|---|---|---|---|---|---|---|---|---|---|---|---|---|---|---|---|---|---|---|---|---|---|---|---|---|
Pronunciation | /ˈɒzmiəm/ | |||||||||||||||||||||||||||||||||||||||||||||||||||||||||||||||||
Appearance | silvery, blue cast | |||||||||||||||||||||||||||||||||||||||||||||||||||||||||||||||||
Standard atomic weight Ar°(Os) | ||||||||||||||||||||||||||||||||||||||||||||||||||||||||||||||||||
Osmium in the periodic table | ||||||||||||||||||||||||||||||||||||||||||||||||||||||||||||||||||
| ||||||||||||||||||||||||||||||||||||||||||||||||||||||||||||||||||
Atomic number (Z) | 76 | |||||||||||||||||||||||||||||||||||||||||||||||||||||||||||||||||
Group | group 8 | |||||||||||||||||||||||||||||||||||||||||||||||||||||||||||||||||
Period | period 6 | |||||||||||||||||||||||||||||||||||||||||||||||||||||||||||||||||
Block | d-block | |||||||||||||||||||||||||||||||||||||||||||||||||||||||||||||||||
Electron configuration | [Xe] 4f14 5d6 6s2 | |||||||||||||||||||||||||||||||||||||||||||||||||||||||||||||||||
Electrons per shell | 2, 8, 18, 32, 14, 2 | |||||||||||||||||||||||||||||||||||||||||||||||||||||||||||||||||
Physical properties | ||||||||||||||||||||||||||||||||||||||||||||||||||||||||||||||||||
Phase at STP | solid | |||||||||||||||||||||||||||||||||||||||||||||||||||||||||||||||||
Melting point | 3306 K (3033 °C, 5491 °F)[3] | |||||||||||||||||||||||||||||||||||||||||||||||||||||||||||||||||
Boiling point | 5281 K (5008 °C, 9046 °F)[4] | |||||||||||||||||||||||||||||||||||||||||||||||||||||||||||||||||
Density (at 20° C) | 22.587 g/cm3[5] | |||||||||||||||||||||||||||||||||||||||||||||||||||||||||||||||||
when liquid (at m.p.) | 20 g/cm3 | |||||||||||||||||||||||||||||||||||||||||||||||||||||||||||||||||
Heat of fusion | 31 kJ/mol | |||||||||||||||||||||||||||||||||||||||||||||||||||||||||||||||||
Heat of vaporization | 378 kJ/mol | |||||||||||||||||||||||||||||||||||||||||||||||||||||||||||||||||
Molar heat capacity | 24.7 J/(mol·K) | |||||||||||||||||||||||||||||||||||||||||||||||||||||||||||||||||
Vapor pressure
| ||||||||||||||||||||||||||||||||||||||||||||||||||||||||||||||||||
Atomic properties | ||||||||||||||||||||||||||||||||||||||||||||||||||||||||||||||||||
Oxidation states | −4, −2, −1, 0, +1, +2, +3, +4, +5, +6, +7, +8 (a mildly acidic oxide) | |||||||||||||||||||||||||||||||||||||||||||||||||||||||||||||||||
Electronegativity | Pauling scale: 2.2 | |||||||||||||||||||||||||||||||||||||||||||||||||||||||||||||||||
Ionization energies |
| |||||||||||||||||||||||||||||||||||||||||||||||||||||||||||||||||
Atomic radius | empirical: 135 pm | |||||||||||||||||||||||||||||||||||||||||||||||||||||||||||||||||
Covalent radius | 144±4 pm | |||||||||||||||||||||||||||||||||||||||||||||||||||||||||||||||||
![]() | ||||||||||||||||||||||||||||||||||||||||||||||||||||||||||||||||||
Other properties | ||||||||||||||||||||||||||||||||||||||||||||||||||||||||||||||||||
Natural occurrence | primordial | |||||||||||||||||||||||||||||||||||||||||||||||||||||||||||||||||
Crystal structure | hexagonal close-packed (hcp) (hP2) | |||||||||||||||||||||||||||||||||||||||||||||||||||||||||||||||||
Lattice constants | ![]() c = 431.99 pm (at 20 °C)[6] | |||||||||||||||||||||||||||||||||||||||||||||||||||||||||||||||||
Thermal expansion | 4.99×10−6/K (at 20 °C)[lower-alpha 1] | |||||||||||||||||||||||||||||||||||||||||||||||||||||||||||||||||
Thermal conductivity | 87.6 W/(m⋅K) | |||||||||||||||||||||||||||||||||||||||||||||||||||||||||||||||||
Electrical resistivity | 81.2 nΩ⋅m (at 0 °C) | |||||||||||||||||||||||||||||||||||||||||||||||||||||||||||||||||
Magnetic ordering | paramagnetic[7] | |||||||||||||||||||||||||||||||||||||||||||||||||||||||||||||||||
Molar magnetic susceptibility | 11×10−6 cm3/mol[7] | |||||||||||||||||||||||||||||||||||||||||||||||||||||||||||||||||
Shear modulus | 222 GPa | |||||||||||||||||||||||||||||||||||||||||||||||||||||||||||||||||
Bulk modulus | 462 GPa | |||||||||||||||||||||||||||||||||||||||||||||||||||||||||||||||||
Speed of sound thin rod | 4940 m/s (at 20 °C) | |||||||||||||||||||||||||||||||||||||||||||||||||||||||||||||||||
Poisson ratio | 0.25 | |||||||||||||||||||||||||||||||||||||||||||||||||||||||||||||||||
Mohs hardness | 7.0 | |||||||||||||||||||||||||||||||||||||||||||||||||||||||||||||||||
Vickers hardness | 4137 MPa | |||||||||||||||||||||||||||||||||||||||||||||||||||||||||||||||||
Brinell hardness | 3920 MPa | |||||||||||||||||||||||||||||||||||||||||||||||||||||||||||||||||
CAS Number | 7440-04-2 | |||||||||||||||||||||||||||||||||||||||||||||||||||||||||||||||||
History | ||||||||||||||||||||||||||||||||||||||||||||||||||||||||||||||||||
Discovery and first isolation | Smithson Tennant (1803) | |||||||||||||||||||||||||||||||||||||||||||||||||||||||||||||||||
Isotopes of osmium | ||||||||||||||||||||||||||||||||||||||||||||||||||||||||||||||||||
| ||||||||||||||||||||||||||||||||||||||||||||||||||||||||||||||||||
Osmium (from Ancient Greek ὀσμή (osmḗ) 'smell') is a chemical element; it has symbol Os and atomic number 76. It is a hard, brittle, bluish-white transition metal in the platinum group that is found as a trace element in alloys, mostly in platinum ores. Osmium is the densest naturally occurring element. When experimentally measured using X-ray crystallography, it has a density of 22.59 g/cm3.[10] Manufacturers use its alloys with platinum, iridium, and other platinum-group metals to make fountain pen nib tipping, electrical contacts, and in other applications that require extreme durability and hardness.[11]
Osmium is among the rarest elements in the Earth's crust, making up only 50 parts per trillion (ppt).[12][13]
Characteristics
Physical properties
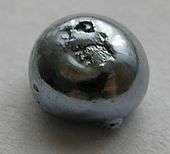
Osmium is a hard, brittle, blue-gray metal, and the densest stable element—about twice as dense as lead. The density of osmium is slightly greater than that of iridium; the two are so similar (22.587 versus 22.562 g/cm3 at 20 °C) that each was at one time considered to be the densest element. Only in the 1990s were measurements made accurately enough (by means of X-ray crystallography) to be certain that osmium is the denser of the two.[10][14]
Osmium has a blue-gray tint.[11] The reflectivity of single crystals of osmium is complex and strongly direction-dependent, with light in the red and near-infrared wavelengths being more strongly absorbed when polarized parallel to the c crystal axis than when polarized perpendicular to the c axis; the c-parallel polarization is also slightly more reflected in the mid-ultraviolet range. Reflectivity reaches a sharp minimum at around 1.5 eV (near-infrared) for the c-parallel polarization and at 2.0 eV (orange) for the c-perpendicular polarization, and peaks for both in the visible spectrum at around 3.0 eV (blue-violet).[15]
Osmium is a hard but brittle metal that remains lustrous even at high temperatures. It has a very low compressibility. Correspondingly, its bulk modulus is extremely high, reported between 395 and 462 GPa, which rivals that of diamond (443 GPa). The hardness of osmium is moderately high at 4 GPa.[16][17][18] Because of its hardness, brittleness, low vapor pressure (the lowest of the platinum-group metals), and very high melting point (the fourth highest of all elements, after carbon, tungsten, and rhenium), solid osmium is difficult to machine, form, or work.
Chemical properties
Oxidation states of osmium | |
---|---|
−4 | [OsIn6−xSnx][19] |
−2 | Na 2[Os(CO) 4] |
−1 | Na 2[Os 4(CO) 13] |
0 | Os 3(CO) 12 |
+1 | OsI |
+2 | OsI 2 |
+3 | OsBr 3 |
+4 | OsO 2, OsCl 4 |
+5 | OsF 5 |
+6 | OsF 6 |
+7 | OsOF 5 |
+8 | OsO 4, Os(NCH 3) 4 |
Osmium forms compounds with oxidation states ranging from −4 to +8. The most common oxidation states are +2, +3, +4, and +8. The +8 oxidation state is notable for being the highest attained by any chemical element aside from iridium's +9[20] and is encountered only in xenon,[21][22] ruthenium,[23] hassium,[24] iridium,[25] and plutonium.[26][27] The oxidation states −1 and −2 represented by the two reactive compounds Na
2[Os
4(CO)
13] and Na
2[Os(CO)
4] are used in the synthesis of osmium cluster compounds.[28][29]
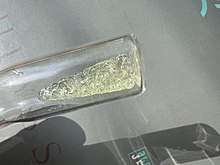
The most common compound exhibiting the +8 oxidation state is osmium tetroxide (OsO4). This toxic compound is formed when powdered osmium is exposed to air. It is a very volatile, water-soluble, pale yellow, crystalline solid with a strong smell. Osmium powder has the characteristic smell of osmium tetroxide.[30] Osmium tetroxide forms red osmates OsO
4(OH)2−
2 upon reaction with a base. With ammonia, it forms the nitrido-osmates OsO
3N−
.[31][32][33] Osmium tetroxide boils at 130 °C and is a powerful oxidizing agent. By contrast, osmium dioxide (OsO
2) is black, non-volatile, and much less reactive and toxic.
Only two osmium compounds have major applications: osmium tetroxide for staining tissue in electron microscopy and for the oxidation of alkenes in organic synthesis, and the non-volatile osmates for organic oxidation reactions.[34]
Osmium pentafluoride (OsF
5) is known, but osmium trifluoride (OsF
3) has not yet been synthesized. The lower oxidation states are stabilized by the larger halogens, so that the trichloride, tribromide, triiodide, and even diiodide are known. The oxidation state +1 is known only for osmium monoiodide (OsI), whereas several carbonyl complexes of osmium, such as triosmium dodecacarbonyl (Os
3(CO)
12), represent oxidation state 0.[31][32][35][36]
In general, the lower oxidation states of osmium are stabilized by ligands that are good σ-donors (such as amines) and π-acceptors (heterocycles containing nitrogen). The higher oxidation states are stabilized by strong σ- and π-donors, such as O2−
and N3−
.[37]
Despite its broad range of compounds in numerous oxidation states, osmium in bulk form at ordinary temperatures and pressures is stable in air. It resists attack by most acids and bases including aqua regia, but is attacked by F2 and Cl2 at high temperatures, and by hot concentrated nitric acid to produce OsO4. It can be dissolved by molten alkalis fused with an oxidizer such as sodium peroxide (Na2O2) or potassium chlorate (KClO3) to give osmates such as K2[OsO2(OH)4].[35]
Isotopes
Osmium has seven naturally occurring isotopes, five of which are stable: 187
Os, 188
Os, 189
Os, 190
Os, and (most abundant) 192
Os. At least 37 artificial radioisotopes and 20 nuclear isomers exist, with mass numbers ranging from 160 to 203; the most stable of these is 194
Os with a half-life of 6 years.[38]
186
Os undergoes alpha decay with such a long half-life (2.0±1.1)×1015 years, approximately 140000 times the age of the universe, that for practical purposes it can be considered stable. 184
Os is also known to undergo alpha decay with a half-life of (1.12±0.23)×1013 years.[9] Alpha decay is predicted for all the other naturally occurring isotopes, but this has never been observed, presumably due to very long half-lives. It is predicted that 184
Os and 192
Os can undergo double beta decay, but this radioactivity has not been observed yet.[38]
189Os has a spin of 5/2 but 187Os has a nuclear spin 1/2. Its low natural abundance (1.64%) and low nuclear magnetic moment means that it is one of the most difficult natural abundance isotopes for NMR spectroscopy.[39]
187
Os is the descendant of 187
Re (half-life 4.56×1010 years) and is used extensively in dating terrestrial as well as meteoric rocks (see Rhenium–osmium dating). It has also been used to measure the intensity of continental weathering over geologic time and to fix minimum ages for stabilization of the mantle roots of continental cratons. This decay is a reason why rhenium-rich minerals are abnormally rich in 187
Os.[40] However, the most notable application of osmium isotopes in geology has been in conjunction with the abundance of iridium, to characterise the layer of shocked quartz along the Cretaceous–Paleogene boundary that marks the extinction of the non-avian dinosaurs 65 million years ago.[41]
History
Osmium was discovered in 1803 by Smithson Tennant and William Hyde Wollaston in London, England.[42] The discovery of osmium is intertwined with that of platinum and the other metals of the platinum group. Platinum reached Europe as platina ("small silver"), first encountered in the late 17th century in silver mines around the Chocó Department, in Colombia.[43] The discovery that this metal was not an alloy, but a distinct new element, was published in 1748.[44] Chemists who studied platinum dissolved it in aqua regia (a mixture of hydrochloric and nitric acids) to create soluble salts. They always observed a small amount of a dark, insoluble residue.[45] Joseph Louis Proust thought that the residue was graphite.[45] Victor Collet-Descotils, Antoine François, comte de Fourcroy, and Louis Nicolas Vauquelin also observed iridium in the black platinum residue in 1803, but did not obtain enough material for further experiments.[45] Later the two French chemists Fourcroy and Vauquelin identified a metal in a platinum residue they called ptène.[46]
In 1803, Smithson Tennant analyzed the insoluble residue and concluded that it must contain a new metal. Vauquelin treated the powder alternately with alkali and acids[47] and obtained a volatile new oxide, which he believed was of this new metal—which he named ptene, from the Greek word πτηνος (ptènos) for winged.[48][49] However, Tennant, who had the advantage of a much larger amount of residue, continued his research and identified two previously undiscovered elements in the black residue, iridium and osmium.[45][47] He obtained a yellow solution (probably of cis–[Os(OH)2O4]2−) by reactions with sodium hydroxide at red heat. After acidification he was able to distill the formed OsO4.[48] He named it osmium after Greek osme meaning "a smell", because of the chlorine-like and slightly garlic-like smell of the volatile osmium tetroxide.[50] Discovery of the new elements was documented in a letter to the Royal Society on June 21, 1804.[45][51]
Uranium and osmium were early successful catalysts in the Haber process, the nitrogen fixation reaction of nitrogen and hydrogen to produce ammonia, giving enough yield to make the process economically successful. At the time, a group at BASF led by Carl Bosch bought most of the world's supply of osmium to use as a catalyst. Shortly thereafter, in 1908, cheaper catalysts based on iron and iron oxides were introduced by the same group for the first pilot plants, removing the need for the expensive and rare osmium.[52]
Osmium is now obtained primarily from the processing of platinum and nickel ores.[53]
Occurrence
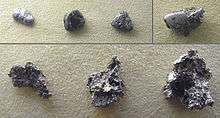
Osmium is one of the least abundant stable elements in Earth's crust, with an average mass fraction of 50 parts per trillion in the continental crust.[54]
Osmium is found in nature as an uncombined element or in natural alloys; especially the iridium–osmium alloys, osmiridium (iridium rich), and iridosmium (osmium rich).[47] In nickel and copper deposits, the platinum-group metals occur as sulfides (i.e., (Pt,Pd)S), tellurides (e.g., PtBiTe), antimonides (e.g., PdSb), and arsenides (e.g., PtAs2); in all these compounds platinum is exchanged by a small amount of iridium and osmium. As with all of the platinum-group metals, osmium can be found naturally in alloys with nickel or copper.[55]
Within Earth's crust, osmium, like iridium, is found at highest concentrations in three types of geologic structure: igneous deposits (crustal intrusions from below), impact craters, and deposits reworked from one of the former structures. The largest known primary reserves are in the Bushveld Igneous Complex in South Africa,[56] though the large copper–nickel deposits near Norilsk in Russia, and the Sudbury Basin in Canada are also significant sources of osmium. Smaller reserves can be found in the United States.[56] The alluvial deposits used by pre-Columbian people in the Chocó Department, Colombia, are still a source for platinum-group metals. The second large alluvial deposit was found in the Ural Mountains, Russia, which is still mined.[53][57]
Production
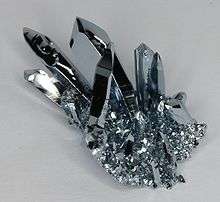
Osmium is obtained commercially as a by-product from nickel and copper mining and processing. During electrorefining of copper and nickel, noble metals such as silver, gold and the platinum-group metals, together with non-metallic elements such as selenium and tellurium, settle to the bottom of the cell as anode mud, which forms the starting material for their extraction.[58][59] Separating the metals requires that they first be brought into solution. Several methods can achieve this, depending on the separation process and the composition of the mixture. Two representative methods are fusion with sodium peroxide followed by dissolution in aqua regia, and dissolution in a mixture of chlorine with hydrochloric acid.[56][60] Osmium, ruthenium, rhodium, and iridium can be separated from platinum, gold, and base metals by their insolubility in aqua regia, leaving a solid residue. Rhodium can be separated from the residue by treatment with molten sodium bisulfate. The insoluble residue, containing ruthenium, osmium, and iridium, is treated with sodium oxide, in which Ir is insoluble, producing water-soluble ruthenium and osmium salts. After oxidation to the volatile oxides, RuO
4 is separated from OsO
4 by precipitation of (NH4)3RuCl6 with ammonium chloride.
After it is dissolved, osmium is separated from the other platinum-group metals by distillation or extraction with organic solvents of the volatile osmium tetroxide.[61] The first method is similar to the procedure used by Tennant and Wollaston. Both methods are suitable for industrial-scale production. In either case, the product is reduced using hydrogen, yielding the metal as a powder or sponge that can be treated using powder metallurgy techniques.[62]
Estimates of annual worldwide osmium production are on the order of several hundred to a few thousand kilograms.[63][35] Production and consumption figures for osmium are not well reported because demand for the metal is limited and can be fulfilled with the byproducts of other refining processes.[35] To reflect this, statistics often report osmium with other minor platinum group metals such as iridium and ruthenium. US imports of osmium from 2014 to 2021 averaged 155 kg annually.[64][65]
Applications
Because osmium is virtually unforgeable when fully dense and very fragile when sintered, it is rarely used in its pure state, but is instead often alloyed with other metals for high-wear applications. Osmium alloys such as osmiridium are very hard and, along with other platinum-group metals, are used in the tips of fountain pens, instrument pivots, and electrical contacts, as they can resist wear from frequent operation. They were also used for the tips of phonograph styli during the late 78 rpm and early "LP" and "45" record era, circa 1945 to 1955. Osmium-alloy tips were significantly more durable than steel and chromium needle points, but wore out far more rapidly than competing, and costlier, sapphire and diamond tips, so they were discontinued.[66]
Osmium tetroxide has been used in fingerprint detection[67] and in staining fatty tissue for optical and electron microscopy. As a strong oxidant, it cross-links lipids mainly by reacting with unsaturated carbon–carbon bonds and thereby both fixes biological membranes in place in tissue samples and simultaneously stains them. Because osmium atoms are extremely electron-dense, osmium staining greatly enhances image contrast in transmission electron microscopy (TEM) studies of biological materials. Those carbon materials otherwise have very weak TEM contrast.[34] Another osmium compound, osmium ferricyanide (OsFeCN), exhibits similar fixing and staining action.[68]
The tetroxide and its derivative potassium osmate are important oxidants in organic synthesis. For the Sharpless asymmetric dihydroxylation, which uses osmate for the conversion of a double bond into a vicinal diol, Karl Barry Sharpless was awarded the Nobel Prize in Chemistry in 2001.[69][70] OsO4 is very expensive for this use, so KMnO4 is often used instead, even though the yields are less for this cheaper chemical reagent.
In 1898, the Austrian chemist Auer von Welsbach developed the Oslamp with a filament made of osmium, which he introduced commercially in 1902. After only a few years, osmium was replaced by tungsten, which is more abundant (and thus cheaper) and more stable. Tungsten has the highest melting point among all metals, and its use in light bulbs increases the luminous efficacy and life of incandescent lamps.[48]
The light bulb manufacturer Osram (founded in 1906, when three German companies, Auer-Gesellschaft, AEG and Siemens & Halske, combined their lamp production facilities) derived its name from the elements of osmium and Wolfram (the latter is German for tungsten).[71]
Like palladium, powdered osmium effectively absorbs hydrogen atoms. This could make osmium a potential candidate for a metal-hydride battery electrode. However, osmium is expensive and would react with potassium hydroxide, the most common battery electrolyte.[72]
Osmium has high reflectivity in the ultraviolet range of the electromagnetic spectrum; for example, at 600 Å osmium has a reflectivity twice that of gold.[73] This high reflectivity is desirable in space-based UV spectrometers, which have reduced mirror sizes due to space limitations. Osmium-coated mirrors were flown in several space missions aboard the Space Shuttle, but it soon became clear that the oxygen radicals in low Earth orbit are abundant enough to significantly deteriorate the osmium layer.[74]
- The Sharpless dihydroxylation:
RL = largest substituent; RM = medium-sized substituent; RS = smallest substituent - A bead of osmium, about 0.5 cm in diameter, displaying the metal's reflectivity
Precautions
The primary hazard of metallic osmium is the potential formation of osmium tetroxide (OsO4), which is volatile and very poisonous.[77] This reaction is thermodynamically favorable at room temperature,[78] but the rate depends on temperature and the surface area of the metal.[79][80] As a result, bulk material is not considered hazardous[79][81][82][83] while powders react quickly enough that samples can sometimes smell like OsO4 if they are handled in air.[35][84]
Price
Between 1990 and 2010, the nominal price of osmium metal was almost constant, while inflation reduced the real value from ~US$950/ounce to ~US$600/ounce.[85] Because osmium has few commercial applications, it is not heavily traded and prices are seldom reported.[85]
Notes
- ↑ The thermal expansion of Os is anisotropic: the coefficients for each crystal axis (at 20 °C) are: αa = 4.57×10−6/K, αc = 5.85×10−6/K, and αaverage = αV/3 = 4.99×10−6/K.
References
- ↑ "Standard Atomic Weights: Osmium". CIAAW. 1991.
- ↑ Prohaska, Thomas; Irrgeher, Johanna; Benefield, Jacqueline; Böhlke, John K.; Chesson, Lesley A.; Coplen, Tyler B.; Ding, Tiping; Dunn, Philip J. H.; Gröning, Manfred; Holden, Norman E.; Meijer, Harro A. J. (May 4, 2022). "Standard atomic weights of the elements 2021 (IUPAC Technical Report)". Pure and Applied Chemistry. doi:10.1515/pac-2019-0603. ISSN 1365-3075.
- ↑ Rumble, John R.; Bruno, Thomas J.; Doa, Maria J. (2022). "Section 4: Properties of the Elements and Inorganic Compounds". CRC Handbook of Chemistry and Physics: A Ready Reference Book of Chemical and Physical Data (103rd ed.). Boca Raton, FL: CRC Press. p. 40. ISBN 978-1-032-12171-0.
- ↑ Rumble, John R.; Bruno, Thomas J.; Doa, Maria J. (2022). "Section 4: Properties of the Elements and Inorganic Compounds". CRC Handbook of Chemistry and Physics: A Ready Reference Book of Chemical and Physical Data (103rd ed.). Boca Raton, FL: CRC Press. p. 40. ISBN 978-1-032-12171-0.
- ↑ Rumble, John R.; Bruno, Thomas J.; Doa, Maria J. (2022). "Section 4: Properties of the Elements and Inorganic Compounds". CRC Handbook of Chemistry and Physics: A Ready Reference Book of Chemical and Physical Data (103rd ed.). Boca Raton, FL: CRC Press. p. 40. ISBN 978-1-032-12171-0.
- ↑ Arblaster, John W. (2018). Selected Values of the Crystallographic Properties of Elements. Materials Park, Ohio: ASM International. ISBN 978-1-62708-155-9.
- 1 2 Haynes 2011, p. 4.134.
- ↑ Kondev, F. G.; Wang, M.; Huang, W. J.; Naimi, S.; Audi, G. (2021). "The NUBASE2020 evaluation of nuclear properties" (PDF). Chinese Physics C. 45 (3): 030001. doi:10.1088/1674-1137/abddae.
- 1 2 Peters, Stefan T.M.; Münker, Carsten; Becker, Harry; Schulz, Toni (April 2014). "Alpha-decay of 184Os revealed by radiogenic 180W in meteorites: Half life determination and viability as geochronometer". Earth and Planetary Science Letters. 391: 69–76. doi:10.1016/j.epsl.2014.01.030.
- 1 2 Arblaster, J. W. (1995). "Osmium, the Densest Metal Known". Platinum Metals Review. 39 (4): 164. doi:10.1595/003214095X394164164. Archived from the original on May 6, 2023. Retrieved November 11, 2023.
- 1 2 Haynes 2011, p. 4.25.
- ↑ Fleischer, Michael (1953). "Recent estimates of the abundances of the elements in the Earth's crust" (PDF). U.S. Geological Survey. Archived (PDF) from the original on October 23, 2022. Retrieved May 10, 2018.
- ↑ "Reading: Abundance of Elements in Earth's Crust | Geology". courses.lumenlearning.com. Archived from the original on May 17, 2022. Retrieved May 10, 2018.
- ↑ Girolami, Gregory (November 2012). "Osmium weighs in". Nature Chemistry. 4 (11): 954. Bibcode:2012NatCh...4..954G. doi:10.1038/nchem.1479. PMID 23089872.
- ↑ Nemoshkalenko, V. V.; Antonov, V. N.; Kirillova, M. M.; Krasovskii, A. E.; Nomerovannaya, L. V. (January 1986). "The structure of the energy bands and optical absorption in osmium" (PDF). Sov. Phys. JETP. 63 (I): 115. Bibcode:1986JETP...63..115N. Archived (PDF) from the original on March 11, 2023. Retrieved December 28, 2022.
- ↑ Weinberger, Michelle; Tolbert, Sarah; Kavner, Abby (2008). "Osmium Metal Studied under High Pressure and Nonhydrostatic Stress". Phys. Rev. Lett. 100 (4): 045506. Bibcode:2008PhRvL.100d5506W. doi:10.1103/PhysRevLett.100.045506. PMID 18352299. S2CID 29146762.
- ↑ Cynn, Hyunchae; Klepeis, J. E.; Yeo, C. S.; Young, D. A. (2002). "Osmium has the Lowest Experimentally Determined Compressibility". Physical Review Letters. 88 (13): 135701. Bibcode:2002PhRvL..88m5701C. doi:10.1103/PhysRevLett.88.135701. PMID 11955108. Archived from the original on September 28, 2023. Retrieved August 27, 2019.
- ↑ Sahu, B. R.; Kleinman, L. (2005). "Osmium Is Not Harder Than Diamond". Physical Review B. 72 (11): 113106. Bibcode:2005PhRvB..72k3106S. doi:10.1103/PhysRevB.72.113106.
- ↑ Fe(−4), Ru(−4), and Os(−4) have been observed in metal-rich compounds containing octahedral complexes [MIn6−xSnx]; Pt(−3) (as a dimeric anion [Pt–Pt]6−), Cu(−2), Zn(−2), Ag(−2), Cd(−2), Au(−2), and Hg(−2) have been observed (as dimeric and monomeric anions; dimeric ions were initially reported to be [T–T]2− for Zn, Cd, Hg, but later shown to be [T–T]4− for all these elements) in La2Pt2In, La2Cu2In, Ca5Au3, Ca5Ag3, Ca5Hg3, Sr5Cd3, Ca5Zn3(structure (AE2+)5(T–T)4−T2−⋅4e−), Yb3Ag2, Ca5Au4, and Ca3Hg2; Au(–3) has been observed in ScAuSn and in other 18-electron half-Heusler compounds. See Changhoon Lee; Myung-Hwan Whangbo (2008). "Late transition metal anions acting as p-metal elements". Solid State Sciences. 10 (4): 444–449. Bibcode:2008SSSci..10..444K. doi:10.1016/j.solidstatesciences.2007.12.001. and Changhoon Lee; Myung-Hwan Whangbo; Jürgen Köhler (2010). "Analysis of Electronic Structures and Chemical Bonding of Metal-rich Compounds. 2. Presence of Dimer (T–T)4– and Isolated T2– Anions in the Polar Intermetallic Cr5B3-Type Compounds AE5T3 (AE = Ca, Sr; T = Au, Ag, Hg, Cd, Zn)". Zeitschrift für Anorganische und Allgemeine Chemie. 636 (1): 36–40. doi:10.1002/zaac.200900421.
- ↑ Stoye, Emma (October 23, 2014). "Iridium forms compound in +9 oxidation state". Chemistry World. Royal Society of Chemistry. Archived from the original on August 9, 2016. Retrieved December 19, 2014.
- ↑ Selig, H.; Claassen, H. H.; Chernick, C. L.; Malm, J. G.; et al. (1964). "Xenon tetroxide – Preparation + Some Properties". Science. 143 (3612): 1322–1323. Bibcode:1964Sci...143.1322S. doi:10.1126/science.143.3612.1322. JSTOR 1713238. PMID 17799234. S2CID 29205117.
- ↑ Huston, J. L.; Studier, M. H.; Sloth, E. N. (1964). "Xenon tetroxide – Mass Spectrum". Science. 143 (3611): 1162–1163. Bibcode:1964Sci...143.1161H. doi:10.1126/science.143.3611.1161-a. JSTOR 1712675. PMID 17833897. S2CID 28547895.
- ↑ Barnard, C. F. J. (2004). "Oxidation States of Ruthenium and Osmium". Platinum Metals Review. 48 (4): 157. doi:10.1595/147106704X10801.
- ↑ "Chemistry of Hassium" (PDF). Gesellschaft für Schwerionenforschung mbH. 2002. Archived from the original (PDF) on January 14, 2012. Retrieved January 31, 2007.
- ↑ Gong, Yu; Zhou, Mingfei; Kaupp, Martin; Riedel, Sebastian (2009). "Formation and Characterization of the Iridium Tetroxide Molecule with Iridium in the Oxidation State +VIII". Angewandte Chemie International Edition. 48 (42): 7879–7883. doi:10.1002/anie.200902733. PMID 19593837.
- ↑ Kiselev, Yu. M.; Nikonov, M. V.; Dolzhenko, V. D.; Ermilov, A. Yu.; Tananaev, I. G.; Myasoedov, B. F. (January 17, 2014). "On existence and properties of plutonium(VIII) derivatives". Radiochimica Acta. 102 (3): 227–237. doi:10.1515/ract-2014-2146. S2CID 100915090.
- ↑ Zaitsevskii, Andréi; Mosyagin, Nikolai S.; Titov, Anatoly V.; Kiselev, Yuri M. (July 21, 2013). "Relativistic density functional theory modeling of plutonium and americium higher oxide molecules". The Journal of Chemical Physics. 139 (3): 034307. Bibcode:2013JChPh.139c4307Z. doi:10.1063/1.4813284. PMID 23883027.
- ↑ Krause, J.; Siriwardane, Upali; Salupo, Terese A.; Wermer, Joseph R.; et al. (1993). "Preparation of [Os3(CO)11]2− and its reactions with Os3(CO)12; structures of [Et4N] [HOs3(CO)11] and H2OsS4(CO)". Journal of Organometallic Chemistry. 454 (1–2): 263–271. doi:10.1016/0022-328X(93)83250-Y.
- ↑ Carter, Willie J.; Kelland, John W.; Okrasinski, Stanley J.; Warner, Keith E.; et al. (1982). "Mononuclear hydrido alkyl carbonyl complexes of osmium and their polynuclear derivatives". Inorganic Chemistry. 21 (11): 3955–3960. doi:10.1021/ic00141a019.
- ↑ Mager Stellman, J. (1998). "Osmium". Encyclopaedia of Occupational Health and Safety. International Labour Organization. pp. 63.34. ISBN 978-92-2-109816-4. OCLC 35279504.
- 1 2 Holleman, A. F.; Wiberg, E.; Wiberg, N. (2001). Inorganic Chemistry (1st ed.). Academic Press. ISBN 978-0-12-352651-9. OCLC 47901436.
- 1 2 Griffith, W. P. (1965). "Osmium and its compounds". Quarterly Reviews, Chemical Society. 19 (3): 254–273. doi:10.1039/QR9651900254.
- ↑ Subcommittee on Platinum-Group Metals, Committee on Medical and Biologic Effects of Environmental Pollutants, Division of Medical Sciences, Assembly of Life Sciences, National Research Council (1977). Platinum-group metals. National Academy of Sciences. p. 55. ISBN 978-0-309-02640-6.
- 1 2 Bozzola, John J.; Russell, Lonnie D. (1999). "Specimen Preparation for Transmission Electron Microscopy". Electron microscopy : principles and techniques for biologists. Sudbury, Mass.: Jones and Bartlett. pp. 21–31. ISBN 978-0-7637-0192-5.
- 1 2 3 4 5 Greenwood, N.N.; Earnshaw, A., eds. (1997). "25 - Iron, Ruthenium and Osmium". Chemistry of the Elements (2 ed.). Butterworth-Heinemann. pp. 1070–1112. doi:10.1016/B978-0-7506-3365-9.50031-6. ISBN 9780750633659.
- ↑ Gulliver, D. J; Levason, W. (1982). "The chemistry of ruthenium, osmium, rhodium, iridium, palladium, and platinum in the higher oxidation states". Coordination Chemistry Reviews. 46: 1–127. doi:10.1016/0010-8545(82)85001-7.
- ↑ Sykes, A. G. (1992). Advances in Inorganic Chemistry. Academic Press. p. 221. ISBN 978-0-12-023637-4.
- 1 2 Kondev, F. G.; Wang, M.; Huang, W. J.; Naimi, S.; Audi, G. (2021). "The NUBASE2020 evaluation of nuclear properties" (PDF). Chinese Physics C. 45 (3): 030001. doi:10.1088/1674-1137/abddae.
- ↑ Bell, Andrew G.; Koźmiński, Wiktor; Linden, Anthony; von Philipsborn, Wolfgang (1996). "187Os NMR Study of (η6-Arene)osmium(II) Complexes: Separation of Electronic and Steric Ligand Effects". Organometallics. 15 (14): 3124–3135. doi:10.1021/om960053i.
- ↑ Dąbek, Józef; Halas, Stanislaw (2007). "Physical Foundations of Rhenium-Osmium Method – A Review". Geochronometria. 27 (1): 23–26. Bibcode:2007Gchrm..27...23D. doi:10.2478/v10003-007-0011-4.
- ↑ Alvarez, L. W.; Alvarez, W.; Asaro, F.; Michel, H. V. (1980). "Extraterrestrial cause for the Cretaceous–Tertiary extinction" (PDF). Science. 208 (4448): 1095–1108. Bibcode:1980Sci...208.1095A. CiteSeerX 10.1.1.126.8496. doi:10.1126/science.208.4448.1095. PMID 17783054. S2CID 16017767. Archived (PDF) from the original on May 21, 2023. Retrieved November 2, 2017.
- ↑ Venetskii, S. I. (1974). "Osmium". Metallurgist. 18 (2): 155–157. doi:10.1007/BF01132596. S2CID 241230590.
- ↑ McDonald, M. (959). "The Platinum of New Granada: Mining and Metallurgy in the Spanish Colonial Empire". Platinum Metals Review. 3 (4): 140–145. doi:10.1595/003214059X34140145. Archived from the original on June 9, 2011. Retrieved October 15, 2008.
- ↑ Juan, J.; de Ulloa, A. (1748). Relación histórica del viage a la América Meridional (in Spanish). Vol. 1. p. 606.
- 1 2 3 4 5 Hunt, L. B. (1987). "A History of Iridium" (PDF). Platinum Metals Review. 31 (1): 32–41. doi:10.1595/003214087X3113241. Archived from the original (PDF) on March 4, 2012. Retrieved March 15, 2012.
- ↑ Haubrichs, Rolf; Zaffalon, Pierre-Leonard (2017). "Osmium vs. 'Ptène': The Naming of the Densest Metal". Johnson Matthey Technology Review. 61 (3): 190. doi:10.1595/205651317x695631.
- 1 2 3 Emsley, J. (2003). "Osmium". Nature's Building Blocks: An A-Z Guide to the Elements. Oxford, England, UK: Oxford University Press. pp. 199–201. ISBN 978-0-19-850340-8.
- 1 2 3 Griffith, W. P. (2004). "Bicentenary of Four Platinum Group Metals. Part II: Osmium and iridium – events surrounding their discoveries". Platinum Metals Review. 48 (4): 182–189. doi:10.1595/147106704X4844.
- ↑ Thomson, T. (1831). A System of Chemistry of Inorganic Bodies. Baldwin & Cradock, London; and William Blackwood, Edinburgh. p. 693.
- ↑ Weeks, M. E. (1968). Discovery of the Elements (7 ed.). Journal of Chemical Education. pp. 414–418. ISBN 978-0-8486-8579-9. OCLC 23991202.
- ↑ Tennant, S. (1804). "On Two Metals, Found in the Black Powder Remaining after the Solution of Platina". Philosophical Transactions of the Royal Society. 94: 411–418. doi:10.1098/rstl.1804.0018. JSTOR 107152. Archived from the original on May 28, 2023. Retrieved August 27, 2019.
- ↑ Smil, Vaclav (2004). Enriching the Earth: Fritz Haber, Carl Bosch, and the Transformation of World Food Production. MIT Press. pp. 80–86. ISBN 978-0-262-69313-4.
- 1 2 George, Micheal W. "2006 Minerals Yearbook: Platinum-Group Metals" (PDF). United States Geological Survey USGS. Archived (PDF) from the original on January 11, 2019. Retrieved September 16, 2008.
- ↑ Wedepohl, Hans K (1995). "The composition of the continental crust". Geochimica et Cosmochimica Acta. 59 (7): 1217–1232. Bibcode:1995GeCoA..59.1217W. doi:10.1016/0016-7037(95)00038-2. Archived from the original on November 3, 2023. Retrieved August 27, 2019.
- ↑ Xiao, Z.; Laplante, A. R. (2004). "Characterizing and recovering the platinum group minerals—a review". Minerals Engineering. 17 (9–10): 961–979. Bibcode:2004MiEng..17..961X. doi:10.1016/j.mineng.2004.04.001.
- 1 2 3 Seymour, R. J.; O'Farrelly, J. I. (2001). "Platinum-group metals". Kirk Othmer Encyclopedia of Chemical Technology. Wiley. doi:10.1002/0471238961.1612012019052513.a01.pub2. ISBN 978-0471238966.
- ↑ "Commodity Report: Platinum-Group Metals" (PDF). United States Geological Survey USGS. Archived (PDF) from the original on January 11, 2019. Retrieved September 16, 2008.
- ↑ George, M. W. (2008). "Platinum-group metals" (PDF). U.S. Geological Survey Mineral Commodity Summaries. Archived (PDF) from the original on January 11, 2019. Retrieved September 16, 2008.
- ↑ George, M. W. 2006 Minerals Yearbook: Platinum-Group Metals (PDF). United States Geological Survey USGS. Archived (PDF) from the original on January 11, 2019. Retrieved September 16, 2008.
- ↑ Renner, H.; Schlamp, G.; Kleinwächter, I.; Drost, E.; et al. (2002). "Platinum group metals and compounds". Ullmann's Encyclopedia of Industrial Chemistry. Wiley. doi:10.1002/14356007.a21_075. ISBN 978-3527306732.
- ↑ Gilchrist, Raleigh (1943). "The Platinum Metals". Chemical Reviews. 32 (3): 277–372. doi:10.1021/cr60103a002. S2CID 96640406.
- ↑ Hunt, L. B.; Lever, F. M. (1969). "Platinum Metals: A Survey of Productive Resources to industrial Uses" (PDF). Platinum Metals Review. 13 (4): 126–138. doi:10.1595/003214069X134126138. Archived from the original (PDF) on October 29, 2008. Retrieved October 2, 2008.
- ↑ Girolami, Gregory (November 2012). "Osmium weighs in". Nature Chemistry. 4 (11): 954. Bibcode:2012NatCh...4..954G. doi:10.1038/nchem.1479. PMID 23089872.
- ↑ Singerling, S.A.; Schulte, R.F. (August 2021). "2018 Minerals Yearbook: Platinum-Group Metals [Advance Release]". Platinum-Group Metals Statistics and Information. U.S. Geological Survey. Archived from the original on July 14, 2023. Retrieved September 24, 2023.
{{cite web}}
: CS1 maint: bot: original URL status unknown (link) - ↑ Schulte, R.F. "Mineral commodity summaries 2022 - Platinum-Group Metals". Platinum-Group Metals Statistics and Information. U.S. Geological Survey. Archived from the original on July 14, 2023. Retrieved September 24, 2023.
{{cite web}}
: CS1 maint: bot: original URL status unknown (link) - ↑ Cramer, Stephen D. & Covino, Bernard S. Jr. (2005). ASM Handbook Volume 13B. Corrosion: Materials. ASM International. ISBN 978-0-87170-707-9.
- ↑ MacDonell, Herbert L. (1960). "The Use of Hydrogen Fluoride in the Development of Latent Fingerprints Found on Glass Surfaces". The Journal of Criminal Law, Criminology, and Police Science. 51 (4): 465–470. doi:10.2307/1140672. JSTOR 1140672. Archived from the original on September 28, 2023. Retrieved December 2, 2018.
- ↑ Chadwick, D. (2002). Role of the sarcoplasmic reticulum in smooth muscle. John Wiley and Sons. pp. 259–264. ISBN 978-0-470-84479-3.
- ↑ Kolb, H. C.; Van Nieuwenhze, M. S.; Sharpless, K. B. (1994). "Catalytic Asymmetric Dihydroxylation". Chemical Reviews. 94 (8): 2483–2547. doi:10.1021/cr00032a009.
- ↑ Colacot, T. J. (2002). "2001 Nobel Prize in Chemistry" (PDF). Platinum Metals Review. 46 (2): 82–83. doi:10.1595/003214002X4628283. Archived from the original (PDF) on January 31, 2013. Retrieved June 12, 2009.
- ↑ Bowers, B., B. (2001). "Scanning our past from London: the filament lamp and new materials". Proceedings of the IEEE. 89 (3): 413–415. doi:10.1109/5.915382. S2CID 28155048.
- ↑ Antonov, V. E.; Belash, I. T.; Malyshev, V. Yu.; Ponyatovsky, E. G. (1984). "The Solubility of Hydrogen in the Platinum Metals under High Pressure" (PDF). Platinum Metals Review. 28 (4): 158–163. doi:10.1595/003214084X284158163. Archived from the original (PDF) on January 31, 2013. Retrieved June 4, 2009.
- ↑ Torr, Marsha R. (1985). "Osmium coated diffraction grating in the Space Shuttle environment: performance". Applied Optics. 24 (18): 2959. Bibcode:1985ApOpt..24.2959T. doi:10.1364/AO.24.002959. PMID 18223987.
- ↑ Gull, T. R.; Herzig, H.; Osantowski, J. F.; Toft, A. R. (1985). "Low earth orbit environmental effects on osmium and related optical thin-film coatings". Applied Optics. 24 (16): 2660. Bibcode:1985ApOpt..24.2660G. doi:10.1364/AO.24.002660. PMID 18223936.
- ↑ Linton, Roger C.; Kamenetzky, Rachel R. (1992). "Second LDEF post-retrieval symposium interim results of experiment A0034" (PDF). NASA. Archived (PDF) from the original on November 4, 2023. Retrieved June 6, 2009.
- ↑ Linton, Roger C.; Kamenetzky, Rachel R.; Reynolds, John M.; Burris, Charles L. (1992). "LDEF experiment A0034: Atomic oxygen stimulated outgassing". NASA Langley Research Center: 763. Bibcode:1992ldef.symp..763L.
- ↑ Lebeau, Alex (March 20, 2015). "Platinum Group Elements: Palladium, Iridium, Osmium, Rhodium, and Ruthenium". Hamilton & Hardy's Industrial Toxicology. John Wiley & Sons, Inc. pp. 187–192. ISBN 978-1-118-83401-5.
- ↑ "Osmium(VIII) oxide". CRC Handbook of Chemistry and Physics, 103rd Edition (Internet Version 2022). CRC Press/Taylor & Francis Group. Archived from the original on October 28, 2023. Retrieved February 6, 2023.
- 1 2 McLaughlin, A. I. G.; Milton, R.; Perry, Kenneth M. A. (July 1, 1946). "Toxic Manifestations of Osmium Tetroxide". Occupational and Environmental Medicine. 3 (3): 183–186. doi:10.1136/oem.3.3.183. PMC 1035752. PMID 20991177.
- ↑ Friedova, Natalie; Pelclova, Daniela; Obertova, Nikola; Lach, Karel; Kesslerova, Katerina; Kohout, Pavel (November 2020). "Osmium absorption after osmium tetroxide skin and eye exposure". Basic & Clinical Pharmacology & Toxicology. 127 (5): 429–433. doi:10.1111/bcpt.13450. PMID 32524772. S2CID 219588237.
- ↑ "Osmium 7440-04-2". Sax's Dangerous Properties of Industrial Materials. John Wiley & Sons, Inc. October 15, 2012. pp. 1–2. doi:10.1002/0471701343.sdp45229. ISBN 978-0-471-70134-7. Retrieved February 5, 2023.
- ↑ Luttrell, William E.; Giles, Cory B. (September 1, 2007). "Toxic tips: Osmium tetroxide". Journal of Chemical Health & Safety. 14 (5): 40–41. doi:10.1016/j.jchas.2007.07.003.
- ↑ Smith, Ivan C.; Carson, Bonnie L.; Ferguson, Thomas L. (August 1974). "Osmium: An Appraisal of Environmental Exposure". Environmental Health Perspectives. 8: 201–213. doi:10.1289/ehp.748201. ISSN 0091-6765. PMC 1474945. PMID 4470919.
- ↑ Gadaskina, I. D. "Osmium". ILO Encyclopaedia of Occupational Health and Safety. Archived from the original on November 3, 2023. Retrieved February 6, 2023.
- 1 2 "USGS Scientific Investigations Report 2012–5188: Metal Prices in the United States Through 2010". pubs.usgs.gov. U.S. Geological Survey. 2013. pp. 119–128. Archived from the original on November 7, 2023. Retrieved July 11, 2023.
Cited sources
- Haynes, William M., ed. (2011). CRC Handbook of Chemistry and Physics (92nd ed.). CRC Press. ISBN 978-1439855119.
External links


- Osmium Archived March 22, 2013, at the Wayback Machine at The Periodic Table of Videos (University of Nottingham)
- Flegenheimer, J. (2014). "The Mystery of the Disappearing Isotope" (via the Wayback Machine). Revista Virtual de Química. V. XX.
- Chisholm, Hugh, ed. (1911). 20 (11th ed.). Cambridge University Press. p. 352. . Encyclopædia Britannica. Vol.